- Division of Quantum Universe
- Division of Photosynthesis and Structural Biology
- Division of Superconducting and Functional Materials
Division of Quantum Universe
Research sector of Atomic Neutrino Spectroscopy
YOSHIMURA Motohiko Group
Taking advantage of the fact that the assumed neutrino mass is close to the energy level spacing of atoms, we are promoting "SPAN (Spectroscopy with Atomic Neutrino)". By using a variety of experimental methods in atomic physics, such as lasers, microwaves, and quantum interference techniques, we aim to observe neutrinos from atoms and determine the unknown parameters of neutrinos. In a recent study, we succeeded in verifying the fundamental part of the "macro-coherence amplification mechanism" by observing coherent two-photon pair emission from a para-hydrogen molecular target transitioned to its oscillation level (v=1) by an adiabatic Raman process.
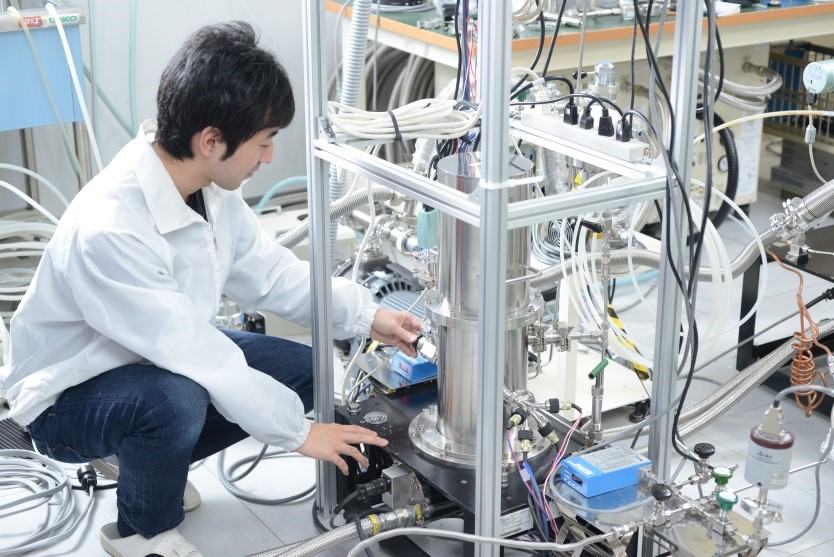
Research Sector of Advanced Quantum Beam
SASAO Group
YOSHIMURA Koji Group
We have been conducting basic research on new frontiers, such as the isomer transition of thorium-229, which has a very low energy for nuclear transitions, and its application (ultra-high-precision nuclear clock), and the unique radiation of coherently excited ion beams accelerated in a magnetic field, We are promoting basic research on new fundamental topics, such as the peculiar radiation when coherently excited ion beams are accelerated in a magnetic field, at the High Brilliance Synchrotron Radiation Facility (SPring-8, High Energy Accelerator Research Organization), the Heavy Ion Source Facility (RIKEN), and the Laser Facility (Okayama University).
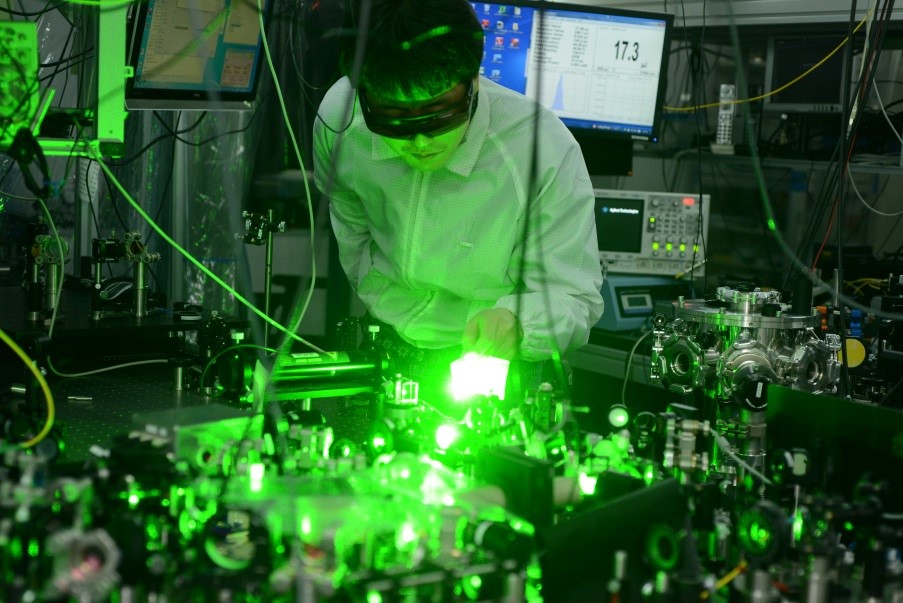
Research Sector of Mathematical Science
TANIGUCHI Group
In Research Sector of Mathematics, Prof. Masaharu Taniguchi studies traveling waves in reaction-diffusion equations, and Prof. Seiichiro Kusuoka studies stochastic differential equations. Many graduate students attend seminars and lectures, and are studying Mathematics everyday.
This picture represents level sets of an axially non-symmetric traveling front (T, SIAM J. Math. Anal. 2015). This traveling front shows that a spatially isotropic reaction-diffusion equation does have axially non-symmetric propagation.
(Figure 1: An axially non-symmetric traveling front)
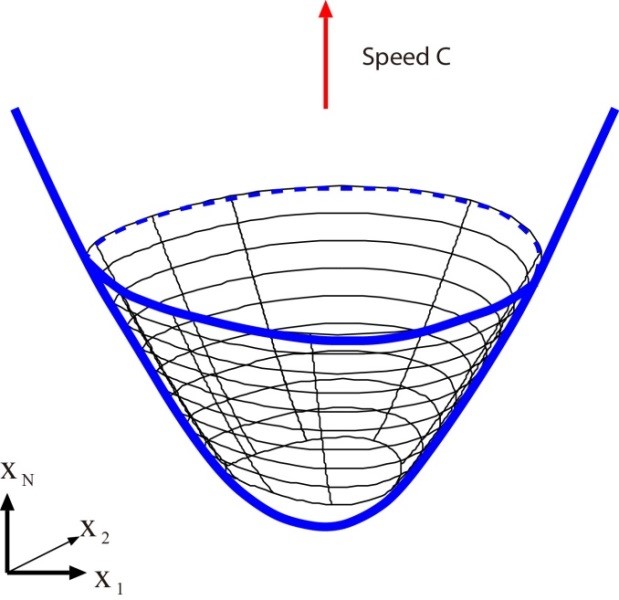
Division of Photosynthesis and Structural Biology
Research Sector of Functional Biology for Photosynthesis
SHEN Group
We study the mechanisms of light-energy absorption, transfer, conversion and water oxidation in natural photosynthesis in various algae and plants, by means of structural biology in combination with a variety of functional approaches. The main targets of our research are photosystem II (PSII) and photosystem I (PSI), two large membrane-protein complexes involved in light energy absorption and conversion, and various light-harvesting pigment antenna systems. PSII utilizes the light-energy to split water, leading to the production of molecular oxygen indispensible for sustaining aerobic life on the earth. We have solved the structure of PSII from a thermophilic cyanobacterium at 1.9 Å resolution (Umena et al. Nature 473, 55-60, 2011), and further obtained its damage-free structure at 1.95 Å resolution (Suga et al. Nature 517, 99-103, 2015) using femtosecond X-ray free electron laser (XFEL) at SACLA, Japan. These studies provided important clues to elucidating the mechanism of light-induced water oxidation. On the other hand, PSI utilizes electrons derived from water via PSII to reduce NADP+, which is then utilized to reduce carbon dioxide into sugars. In higher plants, the core of PSI is surrounded by 4 subunits of light-harvesting complex I (LHCI), forming a PSI-LHCI supercomlex. We have solved the structure of the PSI-LHCI supercomplex from pea at 2.8 Å resolution (Qin et al. Science 348, 989-995, 2015), which provided important information for elucidating the mechanism of highly efficient energy transfer within this supercomlpex.
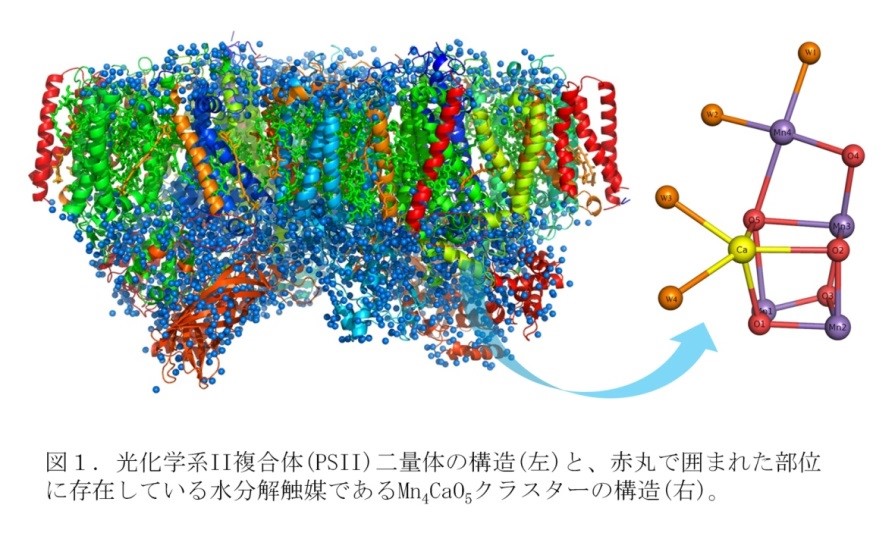
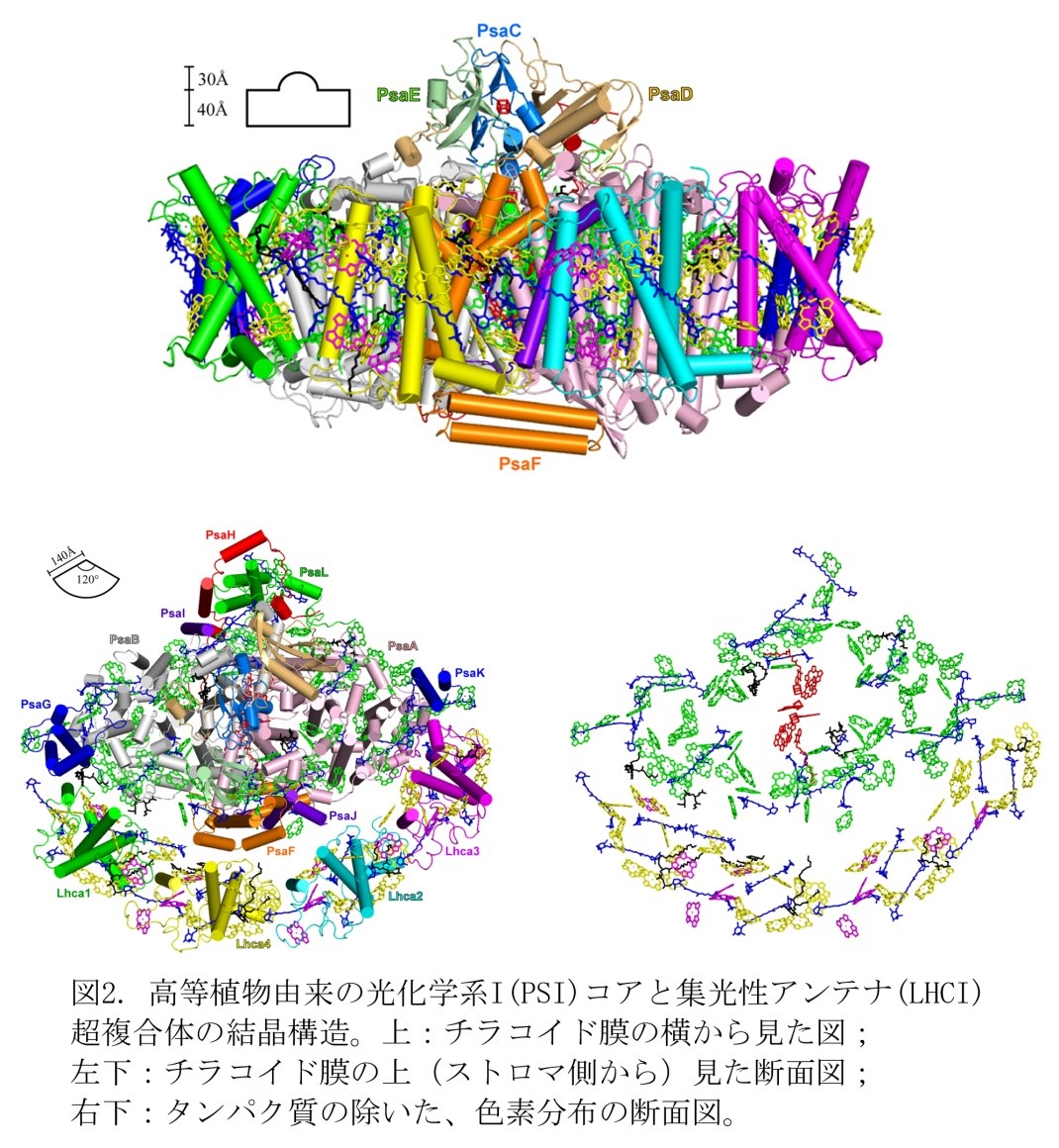
Research Sector of Structural Biology for Photosynthesis
SUGA Group
Research Sector of Artificial Photosynthesis System
SUZUKI Group
New ruthenium(II or III) complexes with the general formula [Ru(O–N)(bpy)2]n+ (O–N = unsymmetrical bidentate phenolate ligand; bpy = 2,2'-bipyridine) were synthesized and their crystal structures and electrochemical properties characterized. The RuII complexes with 2-(2-imidazolinyl)phenolate (Himn–) or 2-(1,4,5,6-tetrahydropyrimidin-2-yl)phenolate (Hthp–) could be deprotonated by addition of excess KOtBu, although the deprotonated species were easily reprotonated by exposure to air. Unlike these RuII complexes, their RuIII analogues showed interesting ligand oxidation reactions upon the addition of bases. With [RuIII(Himn)(bpy)2]2+, two-electron oxidation of Himn– and reduction of the RuIII center resulted in conversion of the 2-imidazolinyl group to a 2-imidazolyl group. On the other hand, the corresponding Hthp– complex exhibited four-electron oxidation of the ligand to form 2-(2-pyrimidyl)phenolate (pym–) (Fig. 1). These aromatization reactions of imidazolinyl and 1,4,5,6-tetrahydropyrimidyl groups were also achieved by the electrochemically generated RuIII complexes.

Fig. 1 Four-electron oxidation of the tetrahydropyrimidyl group induced by oxidation of the Ru center and addition of bases.
Research Sector of Surface Physical Chemistry
YAMAKATA Group
Division of Superconducting and Functional Materials
Research Sector of Physics and Materials Design for High-Tc Superconductors
AKIMITSU Group
Our ultimate goal is to find room temperature superconductors. Superconductor, which holds zero resistance and diamagnetic property, benefits our daily life drastically, if they are realized at room temperature. So far, highest transition temperature (Tc) is below 0 oC and in an extreme environment.
We have been working on synthesizing new multi-functional materials as well as exotic superconductors. Along the way, we have found highest Tc intermetallic compound, MgB2, cuprate superconductor without CuO2 plane, Sr14-xCaxCu24O41 and many more. In the course of finding new high Tc superconductors, we are currently working on light element superconductors and synthesis of metastable material in high pressure/high temperature environment, especially in hydrogen compounds.
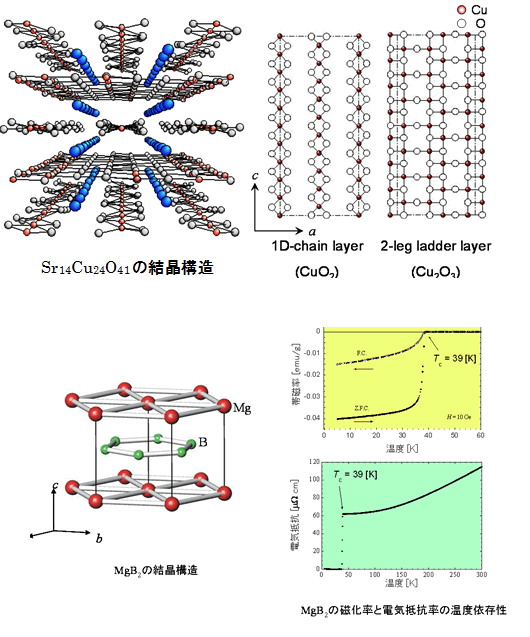
KASAHARA Group
Research Sector of Quantum Physics for Superconductors
YOKOYA Group
The objective of our group is to develop new materials that exhibit useful physical properties (functionalities) and to clarify the origin/mechanism of functionalities. Figure 1 is the bulk sensitive spin -resolved photoemission spectra of high quality CrO2. The combination of high quality CrO2 synthesized in our lab. and the spin-resolved PES constructed in our lab. enables us to observe intrinsic spin-resolved electronic states of CrO2: The electronic states near the Fermi level, which are responsible for the conducting properties, are derived only from electrons with up spin. The result provides reliable spectroscopic evidence for half metallic nature of CrO2. (H. Fujiwara et al., Appl. Phys. Lett. 106, 202404 (2015).)
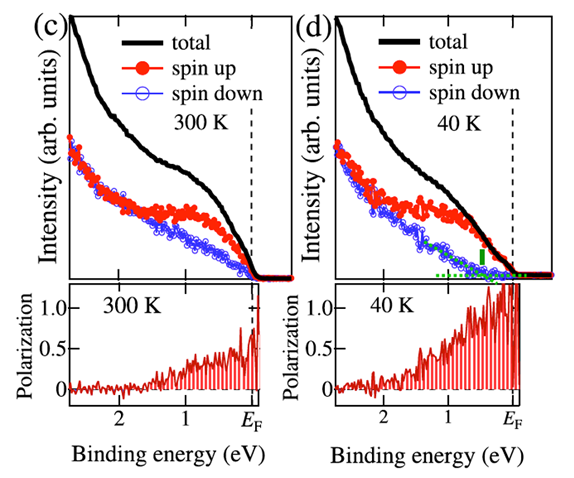
ICHIOKA Group
We (Ichioka, Onari, Adachi) theoretically study condensed matter physics by focusing especially on superconductivity and spin current. Main results in our research are as follows.
(1) We have clarified properties of unconventional superconductivity in the phenomena under magnetic fields (specific heat, magnetization, local electronic states, NMR spectrum, nuclear magnetism relaxation, small angle neutron scattering intensity, etc.) by theoretical calculations of spatial structure in the vortex states.
(2) We study mechanism of superconductivity and transport phenomena in the strongly correlated electron systems. We have clarified that the vertex corrections (higher-order many-body effects) are important in multi-orbital systems such as iron-based superconductors.
(3) We have done a systematic research on the spin Seebeck effect, i.e., the generation of spin currents by thermal energies, and clarified that a low-energy magnetic excitation termed magnon plays an indispensable role in the spin Seebeck effect.
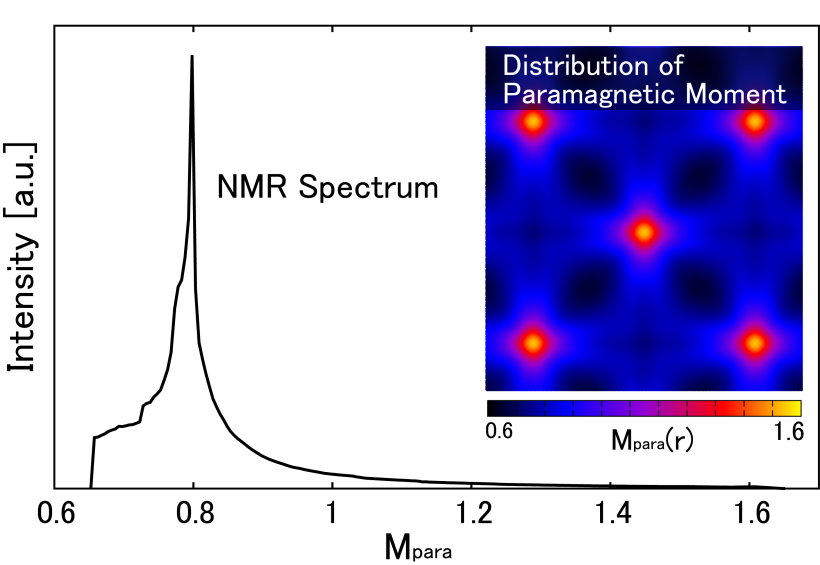
JESCHKE Group
We use density functional theory and manybody methods to study iron pnictide superconductors, quantum spin systems, organic charge transfer salts, metalorganic coordination complexes and strongly correlated oxide materials. The objective of our work is to understand the properties of complex materials and to improve the predictive power of theoretical tools in order to analyze the properties of hypothetical materials designed in the computer. We specially focus on understanding the electronic structure of unconventional superconductors, on energy mapping techniques to work out the Hamiltonian parameters of magnetic materials, on properties of organic and metalorganic complexes in the crystalline state, on the properties of 4d and 5d transition metal compounds with strong spin orbit coupling and on complex oxides with defects.
Research Sector of Light-Element Superconductors and Electronics
KUBOZONO Group
Our group has studied superconductivity induced by chemical doping of two-dimensional (2D) layered materials such as FeSe1-zTez, MoSe2, graphite and polycyclic aromatic hydrocarbon. Recently, pressure-induced high-Tc superconducting phase was discovered in (NH3)yCsxFeSe, which was prepared by intercalating Cs atoms into FeSe in liquid NH3. The (Tc) reached 49 K at 21 GPa (Scientific Reports 5, 9477 (2015)). Furthermore, we have investigated organic field-effect transistors (FETs) using aromatic hydrocarbon such as phenacene molecules. Above all, [9]phenacene single-crystal FETs with ZrO2 gate dielectric showed excellent mobility reaching 18 cm2 V-1 s-1 (Scientific Reports 6<,21008 (2016)). In addition to these topics, electric field control of electronic properties has been intensively studied using 2D layered materials and graphene.
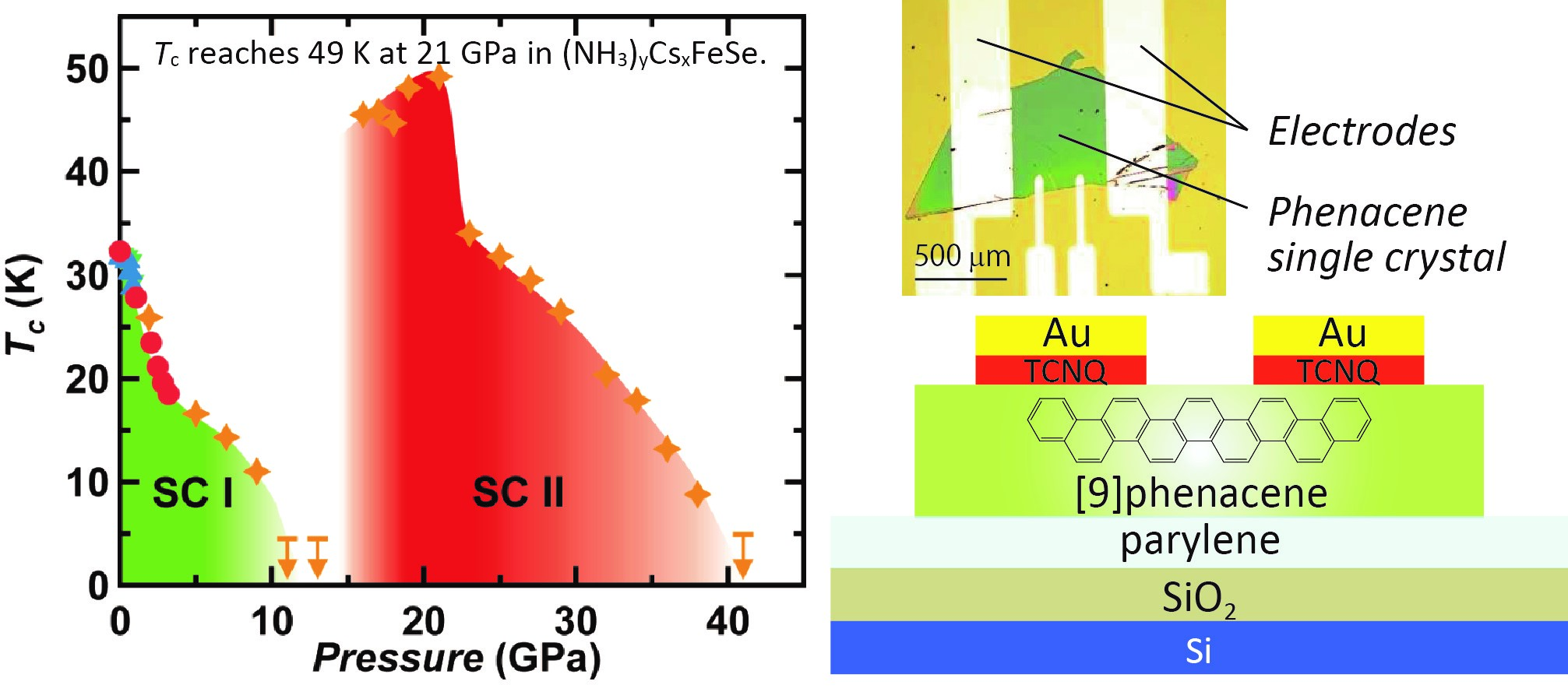
Research Sector of Synthetic Chemistry for Functional Materials
NISHIHARA Group
We have developed efficient synthetic methods and functional organic molecules based on organometallic chemistry. For example, polyaromatic hydrocarbons represent useful compounds for functional organic materials because of their unique redox properties and narrow energy gaps. Thus, we have designed new synthetic methodology to afford the target fused π-electron molecules by using transition metal catalysts, which is more efficient than previously reported conventional synthetic methods. Those compounds are also high potential materials for organic field-effect transistors (OFETs) and organic photovoltaics (OPVs) owing to their strong intermolecular interaction and high carrier transporting ability. By using palladium-catalyzed copolymerization, we have develop new donor-acceptor type semiconducting polymers and high-performance OPV devices.
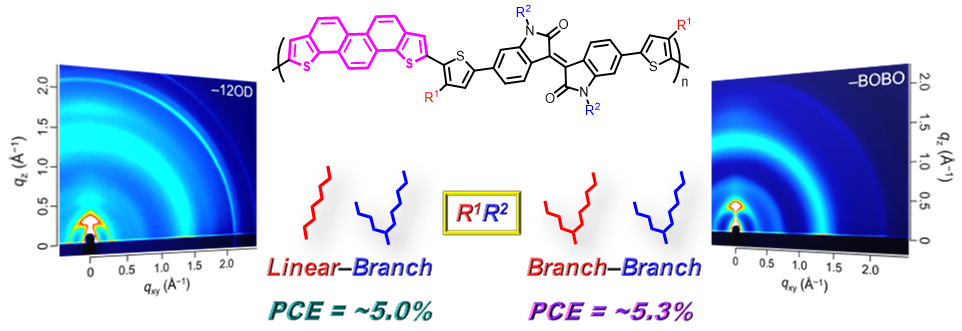
Research Sector of Molecular Science
MATSUMOTO Group
The research interests in our group are mainly focus on exploring phase equilibria and phase transitions between liquids and solids, dynamics and structure of liquids, properties of crystalline and amorphous solids, and surface phenomena, with statistical mechanics, thermodynamics, and computer simulation as the "instrument". Recent studies cover the wide topics including microscopic mechanism of freezing and melting processes of water (recently published in Nature), polyamorphism of supercooled liquid water, water in confined geometry, new polymorph and phase transitions of high-pressure ice, properties of the “forbidden” (i.e. theoretically plausible but inexistent in reality) phases, novel theoretical approach to the stability of clathrate hydrates, and decomposition and formation of clathrate hydrates. These studies are linked to vast research fields such as life sciences, geology and astrogeology, meteorology, and energy resource development.
Recently, we elucidated the adsorption mechanism of the molecules onto the surface of the methane hydrate for the first time by large-scale computer simulations using the K supercomputer and the supercomputers at RCCS. In this study, the molecules are found to be adsorbed on the surface by the entropic force instead of usual energetic stabilization process. (J. Am. Chem. Soc. 2015, 137 (37), 12079–12085.)
- Press release (In Japanese; http://www.okayama-u.ac.jp/tp/release/release_id334.html)
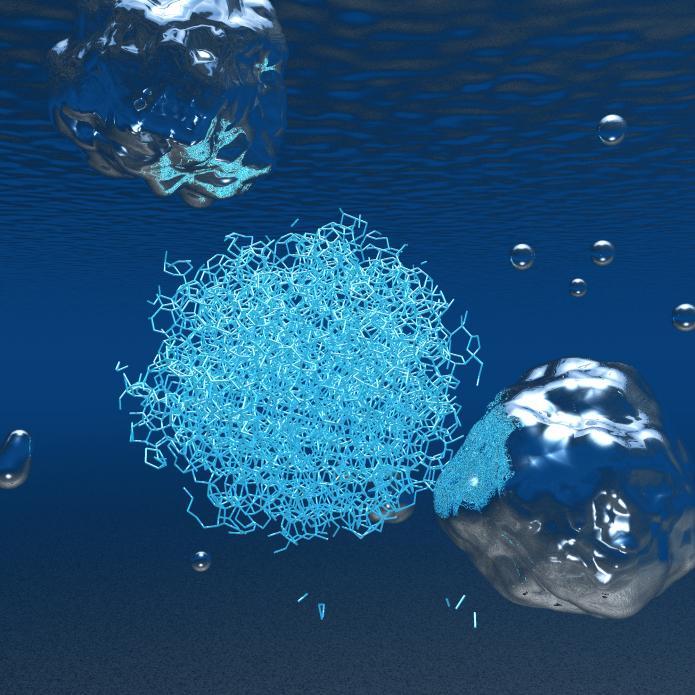
KOGA Group
Phase boundaries between fluids may terminate at the critical point where the two distinct phases lose their identities; Solid-fluid phase boundaries, however, never end at the critical point. This is a common view on the critical point, which has been established by experimental observation and theoretical arguments, but now is no longer as definitive as it was since computer simulations suggested the possibility of solid-liquid critical points for substances confined in nanopores. Recently, we have revisited this problem and have obtained numerical evidence that strongly supports the existence of such critical points for water and argon, both confined in carbon nanotubes [K. Mochizuki and K. Koga, Proc. Natl. Acad. Sci. USA, 112, 8221 (2014)]. There we demonstrated liquid-solid phase equilibria and continuous freezing, located liquid-solid critical points by finite-size scaling analysis, and provided global phase diagrams of water and argon in carbon nanotubes.
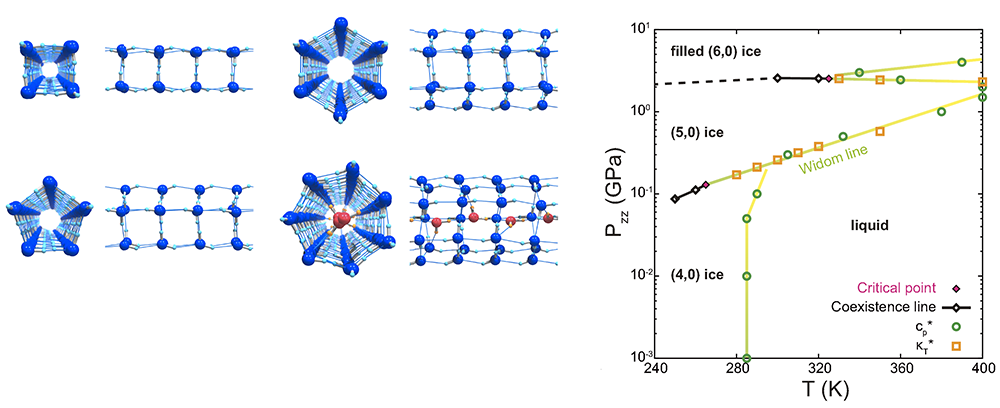
SHINODA Group